4 Ultrasound Bioeffects and Safety
4.1 Learning Objectives
After reviewing this chapter, you should be able to do the following:
- Define the concept of ultrasound bioeffects and describe the various types of bioeffects that can occur during medical ultrasound procedures.
- Explain the physical mechanisms that underlie ultrasound bioeffects, including thermal and nonthermal effects.
- Describe the factors that can influence the likelihood and severity of ultrasound bioeffects, such as the frequency, intensity, and duration of ultrasound exposure.
- Discuss the potential clinical consequences of ultrasound bioeffects, including tissue damage, organ dysfunction, and other adverse outcomes.
- Outline the current safety guidelines and regulations related to medical ultrasound use, including those established by professional organizations and regulatory agencies.
- Identify the key safety considerations and best practices for using ultrasound in clinical practice, including proper equipment calibration, patient positioning, and operator training.
4.2 Introduction
While no medical procedure is entirely risk-free, ultrasound is considered the safest imaging method currently in use. This is because no magnetic field or radiation is used in the imaging process. However, the absence of evidence of harm from any medical procedure does not provide proof of the absence of risks. Therefore, clinicians must take precautions when using any medical technique.
When using ultrasound, risks must be minimized to ensure patient safety. There are two basic rules of medical ethics:[1]
- primum non nocere (translated as “first, do no harm”), and
- perform a procedure only if the importance of doing so outweighs the risk.
In that case, a procedure is deemed ethical only when it benefits the patient.
Ultrasound is generally considered safe if used properly. While there is no evidence it will physically affect the patient long-term, it should still be noted that it is a form of energy, and even at low levels, some studies suggest that overexposure to ultrasound can lead to potential undesirable effects such as
- heat damage to tissue due to energy absorption;
- hearing damage caused by high-frequency sounds from the ultrasound machine; and
- nausea, headaches, and fatigue.
The damage type and extent mainly depend on the ultrasound wave characteristics such as frequency, intensity level, and exposure time, among other factors.
4.3 Acoustic Output Labeling
There are two possible biological effects of ultrasound exposure: (1) thermal (heating) effect and (2) mechanical (cavitation and streaming) effect. These effects can be quantified by reading the mechanical index (MI) and the thermal index (TI) on the ultrasound output display. The MI and TI are on-screen indicators of the potential bioeffects of ultrasound exposure. While imperfect, the TI and MI are considered good thermal and nonthermal risk assessments. These are discussed in detail in the sections below.
4.3.1 Thermal Index
As the ultrasound traverses the tissue, it absorbs some of the energy, causing the tissue temperature to increase. The rate at which the energy is absorbed depends on
- the time of exposure,
- the intensity of the ultrasound beam,
- the attenuation effect of the tissue, and
- the frequency of the ultrasound beam.
Higher thermal effects occur in tissues with higher absorption coefficients (such as bones), and lower thermal effects occur in tissues with lower absorption coefficients (such as amniotic fluids).[2] There is a concern that the embryo and fetus are particularly susceptible to heat energy.
The TI is a relative indicator of thermal risk for the likely temperature rise that might be produced after prolonged exposure. A more significant TI value represents a higher temperature and a higher risk. Three kinds of TI values can be displayed on an ultrasound machine depending on the type of application:[3]
- The TI for soft tissues (TIS) assumes that the ultrasound beam does not impinge on bone, such as is the case for the first trimester.
- The TI for bones (TIB) assumes that the beam impinges on bone at or near its focus, such as is the case for the second and third trimesters.
- The TI for cranial bone (TIC) assumes that the transducer front face is very close to the bone, such as is the case when scanning the adult cranium.
The thermal index (TI) is calculated as the ratio of the acoustic power produced by the transducer (Wp) to the power required by the tissue to raise its temperature by 1 degree Celsius (WDeg). In mathematical form:
The index allows sonographers and clinicians to assess the relative potential heating effects associated with ultrasound imaging. A thermal index of 1 indicates the amount of acoustic power required to raise the tissue temperature by 1 degree Celsius. A higher TI value indicates a higher temperature and a higher risk.
In general, tissue heating by ultrasound is related directly to the intensity of ultrasound waves. The rate of increase in temperature is related to the ultrasound intensity and degree of absorption. It is inversely proportional to tissue density and specific heat. The rate at which the tissue absorbs the heat depends on the protein concentration. For example, fat heats much faster than dense tissue such as muscles.
Nevertheless, the tissue temperature rise is also limited by the cooling effects of the blood flow, which makes it more challenging to heat vascular organs (such as the liver and kidney) than bone.[4] Another concern is that the presence of bones increases the likelihood of a temperature rise due to absorption in the bone and the conduction of heat from bone to adjacent tissues. However, TI values of greater than 1.5 can occur on Doppler ultrasound. Therefore, prolonged pulsed Doppler ultrasound is not recommended for sensitive tissues such as those of the embryo (less than eight weeks), eye, head, brain, and spine.[5]
4.3.2 Mechanical Index
Oscillations of gas bubbles (cavitation) occur inside tissue due to ultrasound pressure waves. The cavitation effect happens due to the excitation of a stable gas bubble by an acoustic field (noninertial cavitation—the formed bubble oscillates in the acoustic field), and streaming effects result from the movement of complex fluids due to radiation force pressures (inertial, or transient, cavitation—the formed bubble rapidly collapses and produces a shock wave that can be capable of causing biological damage).[6],[7] Bubbles are formed in a liquid when the local pressure falls (rarefaction part of the ultrasound wave) below the vapor pressure of the liquid. This cavitation effect is dependent on the fluid inertia, viscosity, and surface tension. Therefore, it is important to shorten the exposure time to minimize cavitation. Another critical point is that cavitation is likely to occur at lower transducer frequencies. However, cavitation-related bioeffects require the presence of cavitation nuclei (or bubbles) close to cells and physical or chemical interaction between bubbles and cells.
The use of the MI as an indicator is based on the assumption that sound induces oscillations of microbubbles, which can cause an increase in the internal temperature of a water gas bubble.
Nevertheless, tissue viscosity is 100 times greater than that of water such that bubble oscillations are greatly limited. A typical display of soft tissue TI and MI values for a carotid exam is shown in the top right corner of the Doppler image in Figure 4-1.
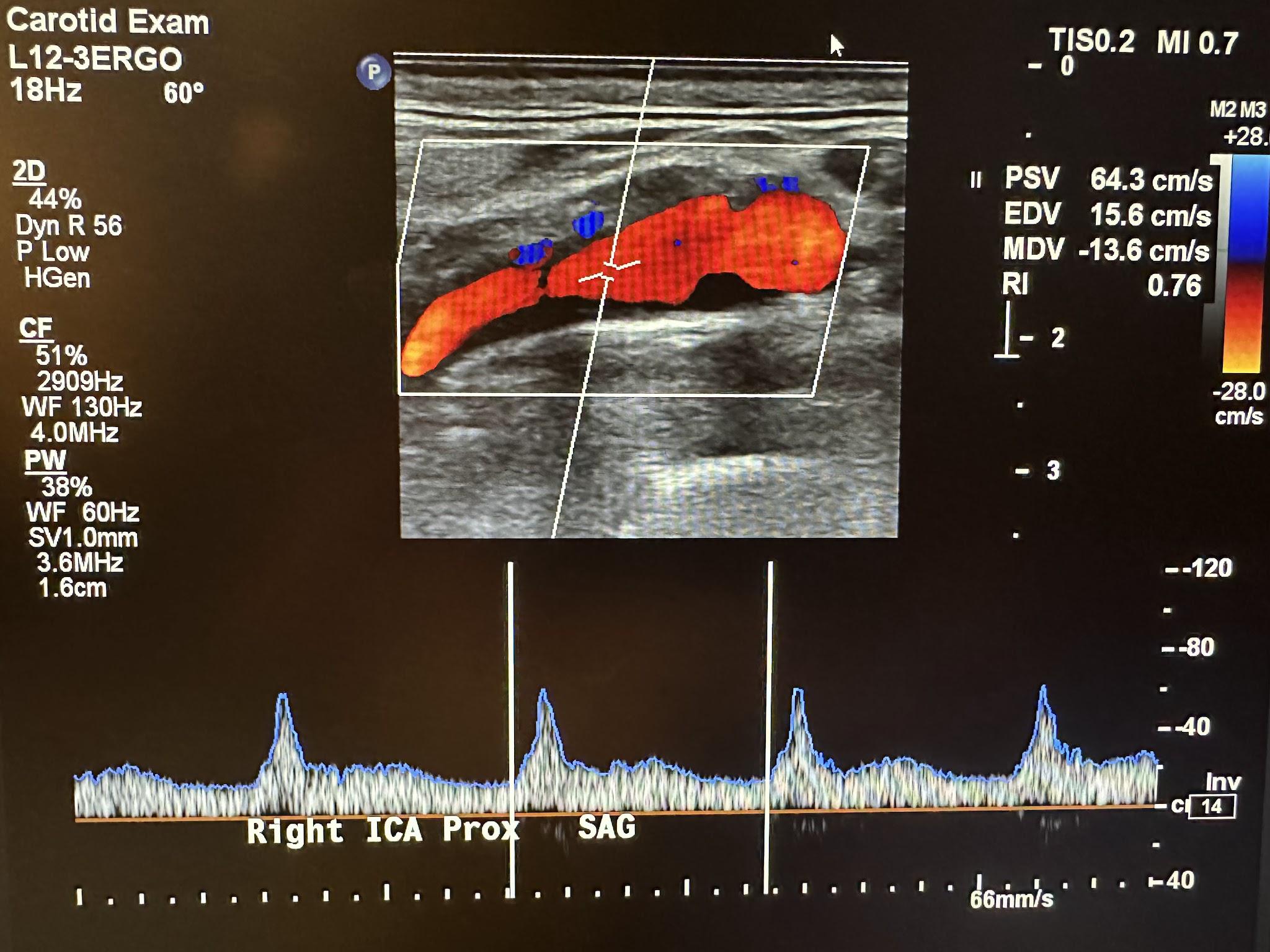
The risk of cavitation increases with increasing MI values. Other studies suggest that high MI values are associated with the induction of premature ventricular contractions in echocardiography.3 The potential for nonthermal biohazards is likely to increase if the equipment is not used correctly. Such biohazards have been observed in animal tissues with gas bubbles at MI values greater than 0.3.[8]3 The bioeffects associated with MI values of 1.9 or less have been reported in skeletal muscle, fat, myocardia, kidneys, livers, and intestines.[9]
Consequently, unnecessary exposure to tissues such as the neonatal lungs should be avoided. Ultrasound operators should keep the MI values as low as possible when carrying out a diagnosis.
4.3.3 Other Indicators
As discussed earlier, the other indicators of thermal effects include the TIS, TIB, and TIC values. The TIS is used when the ultrasound beam passes through soft tissue only, such as in the abdomen and fetal examination during the first trimester. If bones are present, the TIC is used (e.g., in head examinations). The TIB is used if the ultrasound encounters a bone (e.g., in the fetal examination in the second and third trimesters). In addition, the TIS is commonly used for fetal scanning during the first eight weeks of gestation, and the TIB is used after eight weeks. In adults, the TIS is commonly used for eye scanning. In pediatric and adult patients, the TIC is used when examining areas close to skull bone. The TIB is used for all other purposes.
4.4 Potential Electrical and Mechanical Hazards
Following the correct electrical safety procedures reduces the possibility of fire, muscle contracture, and tissue burns. Shocks can occur when a person touches electrical wires due to broken insulation in the circuit, which may contract muscles, alter brain function, or lead to ventricular fibrillation. Small currents can harm patients through saline catheters, which convert them to relatively large current densities. To reduce patient susceptibility to electrical hazards, equipment with any broken or uninsulated cables and damaged probes must not be used.
4.5 Epidemiology
A major concern in the safety of ultrasound has been the exposure of the embryo and fetus during pregnancy examination. Based on current knowledge, there is insufficient information to link diagnostic ultrasound and recognized adverse effects in humans.
4.6 Guidelines and Regulations
The use of diagnostic ultrasound equipment is limited to medical diagnosis only. It can only be used by personnel who are fully trained in the safe and proper operation of the equipment. Other expectations are that the operator should have a complete awareness of machine settings and understand the effect of those machine settings on thermal and mechanical bioeffects. The simple rule that must be followed is that the default power setting protocols should be set at “As Low As (is) Reasonably Achievable” (ALARA) to produce diagnostic quality images. In order to minimize the exposure time on a specific anatomical structure, it is recommended to move or lift the probe when stationary imaging is not necessary. When feasible, it is advisable to avoid imaging fields of view that contain sensitive tissues, including the eye, lungs, and intestines (gas-filled tissues) and fetal calcified structures, such as the skull and spine.
The AIUM, the U.S. Food and Drug Administration (U.S. FDA), and other organizations have issued some guidelines on the use of medical ultrasound in the United States. These guidelines are presented in the sections below.
4.6.1 American Institute of Ultrasound in Medicine Guidelines
The AIUM has issued minimum criteria for a complete medical examination of different parts of the body.[10] According to the AIUM guidelines, there are no independently confirmed significant biological effects in the low megahertz frequency range for ultrasound with intensities below 100 mW/cm2. In addition, for focused ultrasound, such effects have not been proven even at higher intensities for exposure times below 500 s. When adjusting controls that influence acoustic output, the AIUM stresses that it is essential to adhere to the ALARA principle and take into account both the duration of transducer dwell and the total scanning time. It is important to be knowledgeable about the MI upper limit, TI upper limit, and associated duration limits for the examination type being conducted. For example, the guidelines established by Harris et al.[11] and endorsed by the AIUM for the recommended maximum duration of ultrasound exposure at a given setting of the TI are presented in Tables 4-1 and 4-2.
Table 4-1: Recommended maximum exposure time and TI ranges for obstetric (including gynecologic when pregnancy is possible), neonatal transcranial, and neonatal spinal examinations.
TI range |
Time (min) |
>3.0 |
0 |
2.5–3.0 |
<1 |
2.0–2.5 |
<4 |
1.5–2.0 |
<15 |
1.0–1.5 |
<30 |
0.7–1.0 |
<60 |
<0.7 |
No limit |
Table 4-2: Recommended maximum exposure time and TI ranges for adult transcranial, general abdominal, peripheral vascular, neonatal (except head and spine), and other scanning examinations (except the eye).
TI range |
Time (min) |
>6.0 |
0 |
5.0–6.0 |
<0.25 (15 s) |
4.0–5.0 |
<1 |
3.0–4.0 |
<4 |
2.5–3.0 |
<15 |
2.0–2.5 |
<60 |
1.5–2.0 |
<120 |
<1.5 |
No limit |
Even if it is necessary to go beyond the recommended values, an undesirable thermal effect is unlikely to occur in most scanning environments due to attenuating factors such as sensor motion and tissue perfusion. However, it is important to adhere to the ALARA principle so that the duration of the examination is limited to the amount of time needed to obtain a useful diagnostic result.
4.6.2 U.S. Food and Drug Administration Guidelines
Because of the likelihood that ultrasound may have some potential heating and mechanical effects, the U.S. FDA states that the use of ultrasound is not entirely harmless. For this reason, it discourages the nonmedical use of ultrasound devices. The guideline established by the U.S. FDA uses the spatial peak pulse-average intensity (Isppa) and the spatial peak temporal-average intensity (Ispta) in addition to the MI as safety measures for diagnostic ultrasound.[12] Ispta indicates the highest intensity measured at any point in the ultrasound beam averaged over the temporal (time) duration of the pulse, and Isppa represents the highest intensity measured at any point in the ultrasound beam averaged over the pulse repetition period. Table 4-3 lists the highest limit values for these acoustic indicators for diagnostic ultrasound devices.
Table 4-3: U.S. FDA-issued acoustic output exposure level limits.
Use |
Ispta (mW/cm2) |
Isppa (W/cm2) or MI |
Peripheral vessel |
720 |
190 1.9 |
Cardiac |
430 |
190 1.9 |
Fetal imaging and others |
94 |
190 1.9 |
Ophthalmic |
17 |
28 0.23 |
Other categories that could be included in Table 4-3 are abdominal, intraoperative, pediatric, and small organ (breast, thyroid, testes, etc.). The U.S. FDA also mandates the display of the likelihood of ultrasound-induced bioeffects, known as the Standard for Real-Time Display of Thermal and Mechanical Acoustic Output Indices, on diagnostic ultrasound equipment capable of producing higher thermal or mechanical effects. The U.S. FDA discourages any unapproved use of medical devices without a physician’s order. Such practices may be in violation of state laws or regulations. The operator has the ultimate responsibility for the safe use of ultrasound equipment.
4.6.3 Other Professional Societies/Organizations’ Guidelines
The National Collaborating Centre for Women’s and Children’s Health recommends fetal ultrasound screening between 18 and 20 weeks gestation.[13] The guideline was issued on the basis that there is no evidence to support the need for routine use of ultrasound screening after 24 weeks gestation. The Society of Maternal and Fetal Medicine[14] recommended only one medically indicated ultrasound per pregnancy.
4.7 Hygienic Considerations
Medical equipment, including ultrasound machines and transducers, may act as both sources and vectors of microbial transmission during examination. Globally, medical equipment–acquired infections are on the increase. Several studies have confirmed the transmission of bacteria and viruses through improper hygienic use of medical equipment. Common transmittable bacteria and viruses that have been identified include Staphylococcus aureus, Pseudomonas, Acinetobacter species, Candida albicans, hepatitis B, hepatitis C, human immunodeficiency virus, and herpes.[15]
Just as with any medical procedure, there is a potential risk for cross infection (patient-to-patient, patient-to-operator, or operator-to-patient) if proper procedures are not followed. The risk of infection is highest in procedures that utilize transducers in intracavities or where body fluids are encountered. These risks are classified into three categories:
- Critical: Equipment used in settings with a high risk for infection must be sterilized at the time of use. This includes any objects that enter tissue or have been exposed to body fluids.
- Semicritical: Equipment or objects used in procedures in which the items encounter body fluids or nonintact skin should be used once or thoroughly disinfected after every use.
- Noncritical: Equipment that comes in contact with intact skin must be used once and disposed of or sufficiently disinfected after every use.
The cleaning, disinfecting, and sterilizing of reusable medical and surgical equipment or devices must comply with the manufacturer’s requirements and safety protocols. Routine cleaning must include removing the coupling gel and any visible residue from the probe using the recommended detergent. Disposable gloves must be worn during the cleaning process. Areas that require extensive cleaning or disinfection are the transducer probes and ultrasound machine screens. The keyboard is another critical area that can be easily forgotten during cleaning or disinfection. The choice of the keyboard matters—keyboards similar to those used for computers are not ideal for medical purposes, as it is impossible to clean in between the keys after every medical examination. Most modern ultrasound keyboards now come with disinfectable polyurethane covers, which make them easier to clean.
A simple rule is to regard every patient as a potential source of infection. To prevent the risk of cross infection, the following minimum precautions are recommended in every examination:
- Maintain hand hygiene before and after each examination.
- Use personal protective equipment.
- Use clean and disinfected equipment.
- Maintain a clean working environment.
- Correctly dispose of waste.
4.8 Bioethics
While the use of ultrasound in medicine has gained traction over the past two decades, it still faces significant hurdles in some parts of the globe. This is partly due to differences in culture. For example, in some countries, ultrasound is now used for sex-selective abortion.[16] The purpose of this book is not to debate these ethical issues but to bring an awareness of how different societies view the use of ultrasound in medicine.
Clinicians often find themselves in difficult situations, especially when diagnosed anomalies require critical procedures. For example, some fetal abnormalities present ethical dilemmas concerning patient counseling, and abortion issues present challenges in gynecology and obstetrics.
That means clinicians have to play a pivotal role in educating patients about the need to perform these procedures and their benefits to allay the emotional nature of the ultrasound diagnosis or obstetric scanning and the associated implications. In addition, clinicians performing the screening may face dilemmas when diagnosing life-threatening problems with no corrective solutions. In some sections of society, revealing the life span of a patient may not be well received or accepted. That leaves the burden of counseling to the clinicians.
4.9 Self-Assessment
- Why is ultrasound considered relatively safe compared to other imaging equipment?
- What are the two possible biological effects of ultrasound exposure? Briefly discuss them.
- What is the ALARA protocol?
- What are some of the hygienic considerations to remember during and after using ultrasound?
4.10 Further Readings
- American Institute of Ultrasound in Medicine. Recommended maximum scanning times for displayed thermal index (TI) values. Laurel, MD: American Institute of Ultrasound in Medicine website; 2016 Oct 30. Available from: https://www.aium.org/resources/statements.aspx
- American Institute of Ultrasound in Medicine. Medical Ultrasound Safety. 3rd ed. Laurel, MD: American Institute of Ultrasound in Medicine; 2014.
- Quarato CMI, Lacedonia D, Salvemini M, Tuccari G, Mastrodonato G, Villani R, Fiore LA, Scioscia G, Mirijello A, Saponara A, Sperandeo M. A Review on Biological Effects of Ultrasounds: Key Messages for Clinicians. Diagnostics (Basel). 2023 Feb 23;13(5):855. doi: 10.3390/diagnostics13050855. PMID: 36899998; PMCID: PMC10001275.
- Ter Haar G. Ultrasonic imaging: Safety considerations. Interface Focus. 2011 Aug 6;1(4):686–97. doi: 10.1098/rsfs.2011.0029. Epub 2011 May 25. PMID: 22866238; PMCID: PMC3262273. ↵
- Gibbs V, Cole D, Sassano A. Ultrasound Physics and Technology: How, Why and When? [place unknown]: Elsevier Health Sciences; 2009. 144 p. ↵
- Nelson TR, Fowlkes JB, Abramowicz JS, Church CC. Ultrasound biosafety considerations for the practicing sonographer and sonologist. J Ultrasound Med. 2009 Feb;28(2):139–50. doi: 10.7863/jum.2009.28.2.139. PMID: 19168764. ↵
- Ter Haar G. Ultrasonic imaging: Safety considerations. Interface Focus. 2011 Aug 6;1(4):686–97. doi: 10.1098/rsfs.2011.0029. Epub 2011 May 25. PMID: 22866238; PMCID: PMC3262273. ↵
- Nelson TR, Fowlkes JB, Abramowicz JS, Church CC. Ultrasound biosafety considerations for the practicing sonographer and sonologist. J Ultrasound Med. 2009 Feb;28(2):139–50. doi: 10.7863/jum.2009.28.2.139. PMID: 19168764. ↵
- Nelson TR, Fowlkes JB, Abramowicz JS, Church CC. Ultrasound biosafety considerations for the practicing sonographer and sonologist. J Ultrasound Med. 2009 Feb;28(2):139–50. doi: 10.7863/jum.2009.28.2.139. PMID: 19168764. ↵
- Bigelow TA, Church CC, Sandstrom K, Abbott JG, Ziskin MC, Edmonds PD, Herman B, Thomenius KE, Teo TJ. The thermal index: Its strengths, weaknesses, and proposed improvements. J Ultrasound Med. 2011 May;30(5):714–34. doi: 10.7863/jum.2011.30.5.714. PMID: 21527623. ↵
- Nelson TR, Fowlkes JB, Abramowicz JS, Church CC. Ultrasound biosafety considerations for the practicing sonographer and sonologist. J Ultrasound Med. 2009 Feb;28(2):139–50. doi: 10.7863/jum.2009.28.2.139. PMID: 19168764. ↵
- Miller DL, Averkiou MA, Brayman AA, Everbach EC, Holland CK, Wible JH Jr, Wu J. Bioeffects considerations for diagnostic ultrasound contrast agents. J Ultrasound Med. 2008 Apr;27(4):611–32; quiz 633–6. doi: 10.7863/jum.2008.27.4.611. PMID: 18359911. ↵
- American Institute of Ultrasound in Medicine. Medical Ultrasound Safety. 3rd ed. Laurel, MD: American Institute of Ultrasound in Medicine; 2014. ↵
- Harris GR, Church CC, Dalecki D, Ziskin MC, Bagley JE; American Institute of Ultrasound in Medicine; Health Canada; British Medical Ultrasound Society. Comparison of Thermal Safety Practice Guidelines for Diagnostic Ultrasound Exposures. Ultrasound Med Biol. 2016 Feb;42(2):345–57. doi: 10.1016/j.ultrasmedbio.2015.09.016. Epub 2015 Nov 28. PMID: 26626492. ↵
- United States Department of Health and Human Services, United States Food and Drug Administration, United States Center for Devices and Radiological Health. Marketing clearance of diagnostic ultrasound systems and transducers: Guidance for industry and Food and Drug Administration staff. Silver Spring, MD: Center for Devices and Radiological Health; 2019 Jun 27. 60 p. ↵
- National Collaborating Centre for Women’s and Children’s Health (UK). Antenatal Care: Routine Care for the Healthy Pregnant Woman. London: RCOG Press; 2008 Mar. PMID: 21370514. ↵
- Society for Maternal-Fetal Medicine (SMFM), Coding Committee. White paper on ultrasound code 76811. Washington, DC: SMFM; 2004 May 24. Available from: http://www.smfm.org/index.cfm?zone=news&nav=viewnews&newsID=238&smfmon=yes ↵
- Sahu B, Raine-Fenning N. Ultrasound and the risk of nosocomial cross infection. Ultrasound Obstet Gynecol. 2010 Aug;36(2):131–3. doi: 10.1002/uog.7729. PMID: 20681005. ↵
- Tong Y. Changes in son preference, ultrasound use and fertility [internet]. PEW RESEARCH CENTER; 2022 Aug 23. Available from: https://www.ncbi.nlm.nih.gov/books/NBK7274/ ↵